
Euro 2024 will feature the innovative Adidas® Fussballliebe® ball, which boasts a unique and unconventional surface structure with deep circle- and semicircle-shaped ridges and circular dimples, as well as panel seams. When I saw the first pictures of the ball, I knew that we had to model it to get an estimate of its aerodynamic properties. We decided to run a series of simulations and compare the results to the Adidas® Telstar® from the 2018 FIFA World Cup®. Let’s explore our findings…
The Evolution of the New Design
The Euro 2024 tournament kicks off today, June 14, with the Germany-versus-Scotland game in Munich. As usual, Adidas has supplied a new ball for the tournament, the Adidas® Fussballliebe®. The panel design of the Adidas® Fussballliebe® ball seems to be similar to that of the Adidas® Al Rihla® ball, the official ball of the 2022 FIFA World Cup® in Qatar. However, the new ball features a distinct surface design with ridges and dimples. This type of design is also found in the Adidas® MLS 2024 ball and the Adidas® Île-de-Foot 24 ball, the official match ball of the Olympic Games 2024 in Paris.
The Adidas® Fussballliebe® has a very distinct surface design.
In a previous blog series, we analyzed and compared the Adidas® Telstar® ball and the Nike® Ordem V ball. Since then, the panel design of the Adidas® Telstar® ball has been used extensively in a variety of different tournament balls, including the Adidas® MLS Pro 2022 ball; the Adidas® Uniforia® Pro ball, the official match ball of the Euro Cup 2020; and the Adidas® Conext® 21, the official match ball of the 2020 Tokyo Olympic Games.
Since the creation of the Adidas® Telstar®, Adidas has introduced several match ball designs, including the Adidas® Al Rihla® and the Adidas® Oceaunz, which was used in the 2023 FIFA Women’s World Cup. However, the Adidas® Fussballliebe® ball features a design that introduces a major shift compared to these recent Adidas match ball designs. Therefore, it is relevant to compare the Adidas® Telstar® with the Adidas® Fussballliebe® to get a picture of the evolution of the balls used at the highest level of the game and to try to predict the impact of the ball on the outcome of Euro 2024.
Do Any of the Teams Have a Ball Advantage?
The top eight teams favored to win Euro 2024 are ranked in the following order: England, France, Germany, Portugal, Spain, Italy, the Netherlands, and Belgium. They have all practiced and played friendly games with the official ball, although four of the teams are sponsored by Nike and four are sponsored by Adidas.
The top eight teams, showcasing the teams sponsored by Adidas at the top and the teams sponsored by Nike at the bottom.
Interestingly enough, the top eight domestic leagues in Europe also coincide with the top eight ranked national teams at Euro 2024. However, none of these leagues play with Adidas® balls. A large portion of the players in the top eight teams play in the Champions League, where the Adidas® Finale ball is used. However, the Adidas® Finale ball has a substantially different design compared to the Adidas® Fussballliebe®. Players from the Saudi Pro League on the top eight teams of Euro 2024 — such as Aymeric Laporte of Spain, Cristiano Ronaldo and João Neves of Portugal, and Georginio Wijnaldum of the Netherlands — may have a slight advantage, as they are accustomed to playing with the Adidas® Oceaunz, which is similar to the Adidas® Fussballliebe® (although the dimple design differs).
When playing in a tournament, acquaintance with the ball is very important. For example, Diego Forlán, a Uruguayan professional football manager and former player, practiced many hours with the Adidas® Jabulani® before competing in the 2010 FIFA World Cup® in South Africa. Forlán was also among the few players that mastered that ball really well from the beginning of the tournament. He also shared the top scorer position with Thomas Müller, Wesley Sneijder, and David Villa. (All scored 5 goals each.)
All things considered, compared to the total number of players competing in Euro 2024, a relatively small subset of them has experience playing with a ball that is similar to the Adidas® Fussballliebe® — however, the impact of Ronaldo should not be underestimated. Therefore, in terms of match balls, Euro 2024’s ball is relatively fair.
The Complex Shape of the Adidas® Fussballliebe®
The figures below show the geometries of the Adidas® Telstar® and the Adidas® Fussballliebe® used in the simulations in this study. The total seam length for both balls is around 4.3 m. However, the Adidas® Fussballliebe® has a more complex macroscopic surface structure, with ridges, circles, and dimples. Both balls have a microscopic surface texture. We did not include the microscopic surface textures in the 3D CAD models of the geometries.
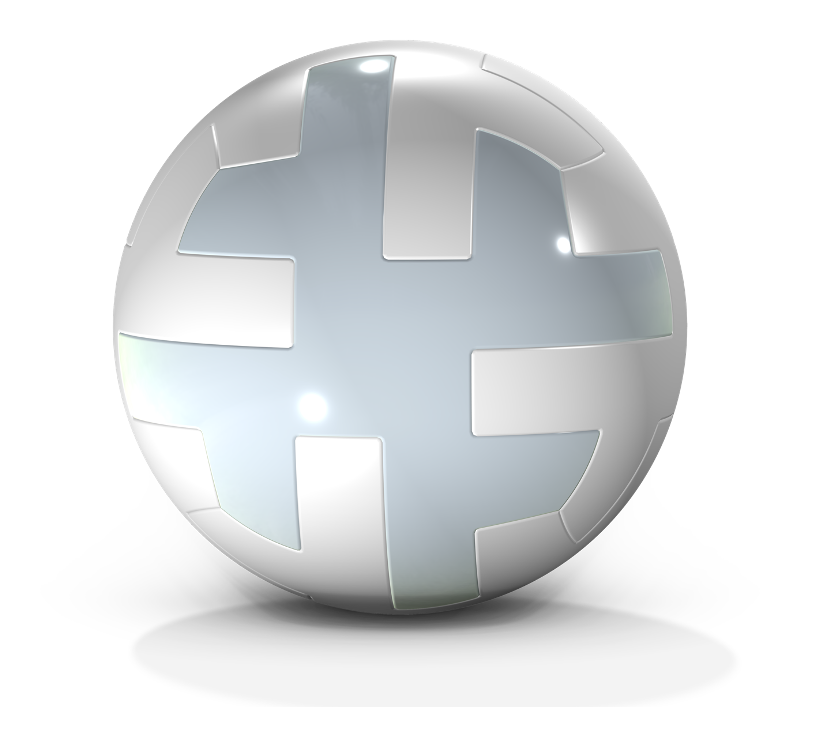
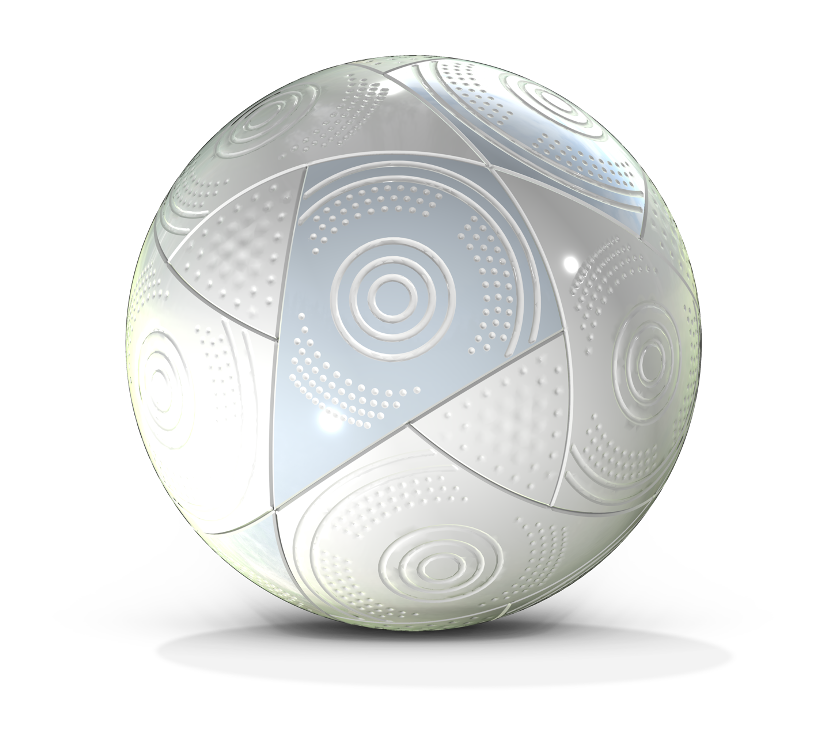
The geometries of the Adidas® Telstar® (left) and the Adidas® Fussballliebe® (right). Note the complex macroscopic surface structure of the Adidas® Fussballliebe®.
Transition from Turbulent to Laminar Boundary Layer
As discussed in our previous blog series, a ball traveling at high speed, for example a free kick, has a turbulent boundary layer (except in a small region surrounding the forward stagnation point) soon after leaving the player’s foot. The turbulent boundary layer squeezes around the ball, with only a small wake zone, giving the ball low drag and a stable flight. As the speed decreases due to drag, the transition from laminar to turbulent boundary-layer flow moves towards the rear, and the detachment eventually occurs in the laminar boundary layer, on the front of the ball. This generates a larger wake behind the ball and, consequently, greater drag and a less stable flight. If this occurs at high velocity and the ball is spinning, then the onset of a stronger Magnus effect is observed. This results in a trajectory that may be straight in the beginning, followed by a sudden curve due to the Magnus effect, as the boundary-layer separation transitions from turbulent to laminar. This was observed in Roberto Carlos’ incredible free kick in the Tournoi de France game between Brazil and France in 1997.
An illustration of Roberto Carlos’ incredible goal for Brazil against France in 1997.
If the ball has no spin, then the straight trajectory is followed by a very unpredictable beach-ball-like trajectory, where the ball may move tens of centimeters (and even meters) to the side of the main trajectory. In addition, if the transition occurs at high speeds, the ball slows down dramatically due to the high drag coefficient for the case of laminar boundary-layer separation. The worst-case scenario for a goal keeper is when the detachment of the boundary layer and the slowdown co-occur, resulting in a slight drop of the ball under the force of gravity. When this happens, a free kick or a shot that appears to go more than a meter above the cross bar may suddenly drop into the goal in the last part of its trajectory.
The dip in drag, induced by the transition from a laminar to turbulent boundary-layer separation, is also referred to as the drag crisis regime. The schematic below shows the drag coefficient as a function of the speed of the ball for different balls.
Schematic for the drag coefficient as a function of the speed of the Adidas® Jabulani® ball (green); the Adidas® Teamgeist® II ball, used in Euro 2008 (blue); and a conventional 32 panel ball, such as the first Adidas® Telstar® from FIFA World Cup® 1970 in Mexico.
The Models: Adidas® Telstar® vs. Adidas® Fussballliebe®
In our study, we used both a large eddy simulation (LES) approach and a Reynolds-averaged Navier–Stokes (RANS) approach, which utilizes a k-ε turbulence model, to analyze and compare the Adidas® Telstar® and the Adidas® Fussballliebe®.
In our first approach, we used the LES method to estimate the drag coefficient of the two balls moving at different speeds. This method is able to capture the transition between laminar and turbulent flow in the boundary layer. As a result, the LES approach provided us with the relative position of the drag crisis due to the macroscopic pattern of the two balls, seams on both balls, and large ridges, grooves, and dimples in the Adidas® Fussballliebe®. Since we did not include the microscopic surface texture in the geometry, we cannot account for its impact on the drag using LES. (Note that the LES method does not include a parameter for surface roughness.)
The mesh with boundary layer mesh used in the LES computations of the Adidas® Fussballliebe®.
In order to capture the impact of the balls’ microscopic surface textures, we introduced parameters for the surface roughness in the k-ε turbulence model. However, this model assumes that the boundary layer is turbulent, so it cannot predict the transition between laminar and turbulent boundary layers. The k-ε turbulence model can tell us the effect of the surface roughness, since we can compare them with the drag coefficient from the LES method.
The Results
The plots below show the velocity field around the Adidas® Telstar® and the Adidas® Fussballliebe®, computed with the LES approach. Both balls are traveling at a speed of 20 m/s, which is well above the drag crisis regime. We can see that the wake region is small, yielding a low drag coefficient, and slightly larger for the Adidas® Fussballliebe®. In addition, the line of separation behind the balls is similar for both balls, although the Adidas® Fussballliebe® seems to have a more stable wake. The animation shows the separation of the boundary layer for the Adidas® Telstar®.
Plots of the velocity field with the magnitude of the velocity vector and streamlines showing the path of the flow around the Adidas® Telstar® and the Adidas® Fussballliebe®.
An animation showing the detachment of the boundary layer on the Adidas® Telstar®, computed with the LES approach.
An animation showing the detachment of the boundary layer on the Adidas® Fussballliebe®, computed with the LES approach.
When traveling at a speed of 20 m/s, the drag coefficient is 0.18 for the Adidas® Telstar® and 0.19 for the Adidas® Fussballliebe®, both computed using LES. This is expected, since the Adidas® Fussballliebe® has an intricate macroscopic surface structure, which may enhance boundary layer transition.
We can compare the drag coefficient for both balls by using the k-ε turbulence model and by assuming a surface roughness coefficient of 0.1 mm (equivalent sand-roughness height). In the plots below, we can deduce that the Adidas® Fussballliebe® has a slightly higher drag coefficient (0.21) compared to the Adidas® Telstar® (0.20). We can also see that the k-ε turbulence model predicts a boundary layer detachment at about the same position compared to the LES results. This is also expected since we are well above the drag crisis, with a turbulent boundary layer in the LES model, and since the k-ε turbulence model assumes a turbulent boundary layer everywhere on the surface of the balls. In addition, the wake region is slightly longer behind the ball compared to the LES results.
Plots of the velocity field with the magnitude of the velocity vector and streamlines showing the path of the flow around the Adidas® Telstar® ball (left) and the Adidas® Fussballliebe® ball (right), computed with a k-ε turbulence model (transient in the case of the Adidas® Telstar®).
The drag coefficient of the Adidas® Telstar® as a function of the speed of the ball shows that the drag crisis for this ball occurs a bit later than a traditional 32-panel ball but earlier than the Adidas® Teamgeist® II. This means it is more stable than the Adidas® Jabulani® and the Adidas® Teamgeist® II. The Adidas Fussballliebe® is expected to be even more stable than the Adidas® Telstar®, and its drag crisis is expected to be only slightly later than that of a traditional 32-panel ball.
The plot below shows the drag coefficient as a function of the speed of the ball using the k-ε turbulence model for the Adidas® Fussballliebe® and LES for the Adidas® Telstar®. Using the k-ε turbulence model, there is no drag crisis, since the model assumes a turbulent boundary layer at all speeds. However, from the preliminary LES for the Adidas® Fussballliebe®, we expect that the the drag crisis will occur at lower speeds. This results in the ball retaining its speed for a longer speed range before being slowed down by laminar boundary-layer separation. The higher drag coefficient at high speed is partly explained by the surface roughness, but, even accounting for the roughness for both balls, the Adidas® Fussballliebe® seems to have a slightly higher drag coefficient than the Adidas® Telstar®.
The Adidas® Telstar® (blue) and the Adidas® Fussballliebe® (green) drag coefficients as a function of the speed of the ball, computed using LES and k-ε turbulence model, respectively.
The Ball Material
One property of the Adidas® Telstar® that stands out is its elasticity, as the elasticity of the ball feels slightly greater than its predecessors at a nominal air pressure. The implication of this is that when the ball is kicked, the ball will move faster and less energy will be dissipated as heat. The Adidas® Fussballliebe® feels even more elastic. (But this is only a subjective feeling when kicking the two balls.)
All in all, we should expect some great goals and hopefully some screamers from 35 m, like Roberto Carlos’ classic goal! And look out for Ronaldo’s free kicks; he will probably master the Adidas® Fussballliebe® better than most players in the tournament.
Stay Tuned for More Updates
A complete study of the Adidas® Telstar® and the Adidas® Fussballliebe® should include the surface texture (the microstructure of the surface) in the CAD model. This would be needed to use LES to get accurate curves for the drag coefficient as a function of the ball speed. In addition, the simulations should be run with different parts of the ball facing the wind to get the natural variations of the drag coefficient. But we only worked on this study for a few days, as it was made for fun and to satisfy our curiosity.
Stay tuned for an update, as we’ll be releasing the results of the Adidas® Fussballliebe® drag coefficient as a function of the ball speed and more once we’ve completed all the necessary computations within this blog post!
Editor’s note: This blog post was updated on June 18, 2024, to reflect updated results.
Dive Into Similar Blog Posts
- Read our other blog posts about the aerodynamics of soccer balls in the following links:
Adidas, Al Rihla, and Fussballliebe are registered trademarks of adidas AG. Jabulani, Teamgeist, and Telstar are registered trademarks of adidas International Marketing B.V.
Nike is a registered trademark of Nike, Inc.
FIFA World Cup is a registered trademark of Federation Internationale de Football Association.
COMSOL AB and its subsidiaries and products are not affiliated with, endorsed, by, sponsored by, or supported by any of the foregoing trademark owners.
Comments (3)
Keyvan Hassanpor
June 18, 2024Good article ,Please post a tutorial about this simulation.
RAJIV KUMAR
June 20, 2024Interesting…thankx
Phillip
June 24, 2024 COMSOL EmployeeI read that 11 of the 34 goals scored on the first day of the European Championship group stage were scored from outside the penalty area – the highest figure at a European Championship and enormous in comparison to the World Cup in Qatar (5%). Have the teams changed their tactics or is this the effect of Fussballliebe’s higher stability? It will be interesting to see whether this trend continues during the tournament…